Competitive Noncovalent Interactions
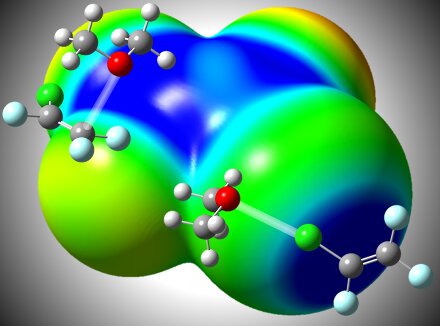
A halogen bond (XB) is a noncovalent interaction between a covalently bonded halogen atom and an electron rich moiety in the same or another molecule. The existence of halogen bonds can be explained theoretically by the presence of an electron deficient region opposite to the covalent bond of the halogen atom, which gives rise to a positive region in the electrostatic potential around the halogen atom in the extension of the covalent bond. This electropositive region, also known as the σ-hole,1 is the result of an anisotropic charge distribution around the halogen atom, where the electrons are concentrated in an ellipsoid around the halogen atom, perpendicular to the covalent bond. Since this noncovalent interaction is quite similar in strength and nature to the ubiquitous hydrogen bonds (HB), 2-4 they can coexist and compete with them. 5-6 Furthermore, when compared to hydrogen bonds, halogen bonds have been found to have greater directionality. 7-8 Therefore, halogen bonds have gained a large increase in interest during the past decade in the fields of supramolecular chemistry and crystal engineering 9 as well as biochemistry, 10 molecular recognition and rational drug design. 11 In order to strengthen these noncovalent interactions, hydrogen and halogen bond donor molecules often contain electron withdrawing groups, such as halogen atoms, or more specifically fluorine atoms. Thanks to the work of Resnati and Metrangolo, perfluorohalocarbons (PFHC’s) are nowadays considered “iconic” halogen bond donors. 12 However, when the locally strongly electron deficient molecules are also unsaturated, they can exhibit a region of positive electrostatic potential perpendicular to the molecular plane. In analogy with the term σ-hole, such a positive region, perpendicular to a portion of the molecular framework, is called a π-hole.13 In recent years, attractive noncovalent interactions between these regions of positive electrostatic potential and electron rich sites in Lewis bases, often called lone pair-π interactions (lp···π), have also been observed in theoretical 14-18 and experimental studies. 19-21
In the TSM² group, we investigate the competition between halogen bonding, hydrogen bonding and lone pair···π interactions experimentally by studying a series of donor molecules which are (theoretically) able to form more than one noncovalent interaction with F, O, N, Cl, S and P containing Lewis bases, as well as π electron donors. To this end, measurements are performed using different techniques, such as infrared and Raman measurements of cryogenic solutions and FT microwave spectroscopy.
Measurements are supported by ab initio calculations, CCSD(T)/CBS energy extrapolations, 22 statistical thermodynamics and Monte Carlo Free Energy Perturbation calculations.6 Furthermore, NCIPlot 23-24 is used to visualize the noncovalent interactions and conceptual DFT is used to rationalize the observed tendencies.
References
1 T. Clark, M. Hennemann, J. S. Murray, P. Politzer, J. Mol. Model., 2007, 13, 291-296.
2 P. Metrangolo, G. Resnati, Science, 2008, 321, 918-919.
3 P. Politzer, J. S. Murray, ChemPhysChem, 2013, 14, 278-294.
4 Z. P. Shields, J. S. Murray, P. Politzer, International Journal of Quantum Chemistry, 2010, 110, 2823-2832.
5 P. Politzer, J. S. Murray, P. Lane, International Journal of Quantum Chemistry, 2007, 107, 3046-3052.
6 N. Nagels, Y. Geboes, B. Pinter, F. De Proft, W. A. Herrebout, Chem. - Eur. J., 2014, 20, 8433-8443.
7 P. Politzer, J. S. Murray, T. Clark, Phys Chem Chem Phys, 2010, 12, 7748-7757.
8 R. Wilcken, M. O. Zimmermann, A. Lange, A. C. Joerger, F. M. Boeckler, J. Med. Chem., 2012, 56, 1363-1388.
9 P. Metrangolo, G. Resnati, T. Pilati, S. Biella, Struct. Bond., 2008, 126, 105-136.
10 M. R. Scholfield, C. M. Zanden, M. Carter, P. S. Ho, Protein Sci., 2013, 22, 139-152.
11 S. Sirimulla, J. B. Bailey, R. Vegesna, M. Narayan, J. Chem. Inf. Model., 2013, 53, 2781-2791.
12 R. W. Troff, T. Mäkelä, F. Topić, A. Valkonen, K. Raatikainen, K. Rissanen, Eur. J. Org. Chem., 2013, 2013, 1617-1637.
13 J. S. Murray, P. Lane, T. Clark, K. E. Riley, P. Politzer, J. Mol. Model., 2012, 18, 541-548.
14 B. W. Gung, Y. Zou, Z. G. Xu, J. C. Amicangelo, D. G. Irwin, S. Q. Ma, H. C. Zhou, J. Org. Chem., 2008, 73, 689-693.
15 T. Korenaga, T. Shoji, K. Onoue, T. Sakai, Chem. Commun., 2009, 4678-4680.
16 I. Alkorta, I. Rozas, M. L. Jimeno, J. Elguero, J. Struct. Chem., 2001, 12, 459-464.
17 I. Alkorta, I. Rozas, J. Elguero, J. Org. Chem., 1997, 62, 4687-4691.
18 M. Egli, S. Sarkhel, Acc. Chem. Res., 2007, 40, 197-205.
19 T. J. Mooibroek, P. Gamez, J. Reedijk, CrystEngComm, 2008, 10, 1501-1515.
20 J. C. Amicangelo, D. G. Irwin, C. J. Lee, N. C. Romano, N. L. Saxton, J. Phys. Chem. A., 2013, 117, 1336-1350.
21 N. Ma, Y. Zhang, B. Ji, A. Tian, W. Wang, ChemPhysChem, 2012, 13, 1411-1414.
22 Y. Geboes, N. Nagels, B. Pinter, F. De Proft, W. A. Herrebout, J. Phys. Chem. A, 2015, 119, 2502–2516.
23 J. Contreras-Garcia, E. R. Johnson, S. Keinan, R. Chaudret, J. P. Piquemal, D. N. Beratan, W. Yang, J. Chem. Theory Comput., 2011, 7, 625-632.
24 E. R. Johnson, S. Keinan, P. Mori-Sanchez, J. Contreras-Garcia, A. J. Cohen, W. T. Yang, J. Am. Chem. Soc., 2010, 132, 6498-6506.