CO2 conversion into value-added chemicals and renewable fuels is one of the great challenges of the 21stcentury, as it might contribute to a solution for the problem of global warming and it also reduces our dependence on fossil fuels. Plasma technology is very promising as energy-efficient alternative to the existing classical conversion methods, because the splitting of the inert molecules is initiated by energetic electrons present in the plasma. Moreover, plasma uses electricity and can easily be switched on/off, so it has great potential for temporary storage of excess renewable energy, which is also considered as one of the major challenges of this century. Thus, plasma has the potential to simultaneously solve 3 major problems: (i) global warming, (ii) our dependence on fossil fuels, and (iii) the transition to renewable energy. In addition, by combining the plasma with a catalyst, in so-called “plasma catalysis”, the selective production of value-added chemicals can be targeted. However, more fundamental research is crucially needed, to better understand the underlying mechanisms, in order to improve the conversion and energy efficiency of plasma technology. For this reason, we perform computer modelling as well as experiments. We model the plasma chemistry by 0D chemical kinetics modelling, focussing among others on the role of CO2 vibrational levels versus thermal conversion, as well as on mixtures of CO2 with CH4, H2O, H2 and N2. Furthermore, we also model various plasma reactors (i.e., dielectric barrier discharges (DBDs) and packed bed DBDs, microwave plasmas, gliding arc and atmospheric pressure glow discharges) by fully coupled 3D-axisymmetric and 2D-fluid dynamics models, to improve the design for energy-efficient CO2 conversion and investigate the effect of post-plasma gas quenching. Finally, we also model plasma-catalyst interaction (i.e., penetration of plasma species inside catalyst pores, and the chemical reactions at the catalyst surface. Our experiments are performed in various types of plasma reactors: cylindrical gliding arc plasmas (gliding arc plasmatron with reverse vortex flow, rotating gliding arc, …), microwave plasmas, atmospheric pressure glow discharges, pin-to-pin arc plasmas, and (packed bed) DBDs.
Moreover, besides CO2 conversion, plasma is also gaining increasing interest for CH4 conversion into either H2 (and value-added carbon) or into olefins, like ethylene (C2H4) and acetylene (C2H2), which are of great interest for the chemical industry. In addition, plasma can also be important for H2 synthesis from other hydrocarbons (e.g., methanol, ethanol, and even plastic waste pyrolysis products), as well as from NH3. We also perform research for these applications, again by a combination of plasma chemistry and plasma reactor modeling, and experiments.
Finally, plasma is also very promising for nitrogen fixation, i.e., the conversion of nitrogen from the atmosphere into chemical building blocks for all living organisms. This is a very important process in sustainable chemistry. Indeed, nitrogen is needed for the biosynthesis of all basic building blocks of plants and living organisms, such as amino acids, peptides and nucleic acids. There is more than enough nitrogen present in our atmosphere for this purpose, but these N2 molecules are very stable, and a lot of energy is required to break the triple bond between the N-atoms. Hence, it is important to develop energy-efficient processes for nitrogen fixation, where nitrogen from the atmosphere is converted into simple nitrogen compounds, like ammonia (NH3) or nitrogen oxides (NO, NO2), which can more easily be used as precursors for the biosynthesis of more complex molecules. Plasma is gaining increasing interest for the activation of nitrogen molecules in an energy-efficient way. Moreover, as mentioned above, plasma can be used for the storage of excess renewable energy, and it is very modular. Hence, this process also has great potential to be used at remote locations, for the local production of fertilizers by means of plasma, simply using air, in combination with solar or wind energy. However, more research is needed to further improve the energy efficiency of this process. Again, we are developing models and performing experiments in various types of plasma reactors, for better insight and improving the performance.
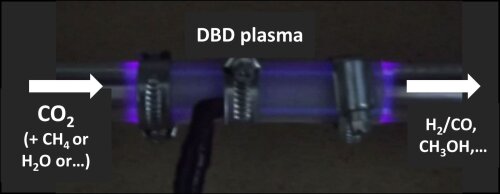